1.IntroductionEpithelial ovarian cancer has the highest mortality rate of any of the gynecologic cancers. Only 40 of patients survive five years, despite aggressive treatment, due, in part, to the fact that 70 of patients are diagnosed after metastases have already occurred.1 Given our inability to cure ovarian cancer, strategies for prevention merit at least as much attention as does treatment of disease. Cancer chemoprevention refers to the administration of chemical agents that prevent or delay the development of cancer in healthy people. Biomarkers that are likely to be affected by the preventive agent and whose modulation supports the postulated chemopreventive activity2 3 are one of the most important components for prevention studies. Identification of these predictive biomarkers would shorten the time necessary for prevention studies by assessing drug activity rather than following patients for the years that it takes for cancers to develop.3 However, development of biomarkers is the most difficult and time consuming aspect of prevention studies and usually requires an invasive biopsy. The ability to noninvasively monitor drug activity wold be a major advancement in the prevention of ovarian cancer and could potentially be extended to other organ sites, thus reducing the morbidity of preventing disease. Retinoids, vitamin A derivatives, have been studied as cancer chemopreventive agents4 5 6 7 based on epidemiologic data showing that diets high in vitamin A are associated with lower odds of epithelial cancers. An Italian trial that evaluated N-(4-hydroxyphenyl) retinamide (4-HPR) for prevention of secondary breast cancers incidentally demonstrated a decreased incidence of ovarian cancer in the women receiving 4-HPR, suggesting that retinoids prevented the development of ovarian cancer.4 After cessation of 4-HPR treatment, new ovarian cancers occurred in the treatment group, suggesting that the prevention was not durable. Experimental studies have demonstrated that retinoids can affect human ovarian cancer cell growth by inhibiting proliferation and inducing apoptosis,5 6 7 which are thought to be important mechanisms in cancer prevention.8 Retinoids, particularly 4-HPR, have been shown to increase aerobic glycolysis by increasing mitochondrial permeability to the co-enzymes nicotinamide adenine dinucleotide (NAD(P)H) and flavin adenine dinucleotide (FAD), as well as activity of the electron transport chain characterized by an increase in reactive oxygen species and cytochrome oxidase.9 10 Thus, retinoids are drugs whose activities are potentially amenable to surveillance with fluorescence spectroscopy. Other chemopreventive agents are also of interest in the ovary. Multiple epidemiologic studies have shown that the oral contraceptive pill (OCP) use for at least five years is associated with a 50 or greater reduction in the odds of developing ovarian cancer.11 12 13 14 15 The mechanism of this prevention is unclear; one factor may be suppression of ovulation, but other mechanisms are hypothesized. A single prospective study has shown that OCP and progesterone increased the rate of apoptosis of ovarian surface epithelial cells in primates.16 In the last decade, there has been substantial research to develop optical methods as early diagnostic tools for cancers.17 18 19 20 27 Current diagnostic techniques lack sufficient predictive value to diagnose preinvasive cancers when they might be prevented from progressing or to diagnose small invasive cancers that might be cured with minimal morbidity. Novel optical techniques can aid in the early, near real-time diagnosis by localizing abnormal areas without a visible lesion for biopsy. This paper describes the use of fluorescence spectroscopy as a marker for the action of drugs that may prevent cancers by inducing quiescence of those cells destined to develop into cancer or by reversing preinvasive changes that have not yet developed into a cancer. Natural fluorophores in tissue include NAD(P)H, FAD, structural proteins such as collagen, elastin, and their cross-links, and the aromatic amino acids tryptophan, tyrosine, and phenylalanine, each of which has a characteristic wavelength for excitation with an associated characteristic emission. Fluorescence collected on the tissue surface is also affected by absorption and scattering. Fluorescence spectroscopy is being used to detect cancers noninvasively in many organ systems, including the cervix, head, and neck and the lungs.17 18 19 20 Fluorophore concentrations change as normal tissues progress to cancer.21 Different changes in the fluorescence signature may occur in response to an agent that arrests cell growth or induces apoptosis. In this study, we evaluated fluorescence spectroscopy as a marker for drug activity in the ovary in the monkey and as a measure of metabolic activity in cell culture. A redox ratio can be calculated as the ratio of the FAD fluorescence intensity to that of the sum of FAD and NAD(P)H intensities, and is a measure of aerobic glycolysis in tissue.21 An increasing redox ratio signifies that either FAD fluorescence intensity has increased, NAD(P)H fluorescence intensity has decreased, or both changes have occurred. In a prior pilot study in women,22 differences in excitation-emission matrices (EEMs) from normal ovaries and invasive cancer were observed with peaks at 350 nm excitation and 460 nm emission wavelengths that represent both collagen and NAD(P)H. These peaks were higher in the cancers suggesting increased NAD(P)H signal. In tumors, these co-enzymes are thought to exist in their reduced state (NAD(P)H,FADH) with a unique fluorescence signature (NAD(P)H high, FAD low) as a result of alterations in blood flow, decreased pH of the tissue, abnormal mitochondria, and abnormal transport of electron carrier molecules into the mitochondria21 where the electron transport chain takes place. This study is a feasibility study to explore fluorescence spectroscopy as a biomarker for drug activity. In the present study we have used three different human ovarian epithelial cell lines to explore whether apoptosis and growth inhibition induced by 4-HPR are correlated with changes in NAD(P)H and FAD fluorescence intensity and the resultant redox potentials. We have further explored the changes in fluorescence spectra in response to chemopreventive agents in a primate model that allows us to evaluate the response of the ovary in situ to these drugs where the surface epithelial cells are in contact with stromal cells. The cell model has permitted evaluation of several different combinations and doses of drugs, whereas the primate provides an in vivo model that can provide a bridge for human clinical studies due to similarities in reproductive function between primates and women. These studies have permitted us to evaluate the potential role of fluorescence spectroscopy as a biomarker for chemopreventive drug activity in the ovary. 2.Materials and Methods2.1.Cell Culture2.1.1.Experimental DesignThree different cell lines were incubated with different doses of 4-HPR (0, 2, 5, 10 μM) to evaluate sensitivity to 4-HPR. TGF-β1 has been shown to have additive effects in some cell lines in the induction of apoptosis, but had no effect in these cultures. Primary cultures of normal ovarian epithelial (NOE) cells were established from surgical specimens of normal ovaries as previously described.23 They were maintained in a 1:1 mixture of cell culture media MCDB 105 and Medium 199 supplemented with 5 fetal bovine serum, 2 mM L-glutamine, 100 units/ml penicillin and 100 μg/mL streptomycin. Simian virus 40-immortalized ovarian surface epithelium cell lines IOSE-29 and IOSE-261 were established as immortalized cell lines. They were maintained in a 1:1 mixture of MCDB 105 and Medium 199 supplemented with 5 fetal bovine serum and 50 μg/mL gentamycin sulfate. 2.1.2.FluorescenceNOE, IOSE 29, and IOSE 261 were the three cell lines used. After three days of treatment with 4-HPR or diluent, cells were harvested. Since the media itself shows a strong auto-fluorescence the cells were washed three times with phosphate buffered saline (PBS) before optical studies were performed. For the spectroscopic studies, all cells were diluted in sterile buffered isotonic saline solution (PBS) with 5 glucose. The cell suspension was centrifuged at 400 g for 10 min. then washed three times. Fluorescence emission was measured using a scanning spectrofluorimeter (Hitachi Ltd., F-4010, Tokyo, Japan). Excitation light was generated at three wavelengths to probe for fluorescence consistent with tryptophan, the co-factors NAD(P)H and FAD. Because of the design of the fluorimeter and the inherently weak signal of the autofluorescence of the cell suspension, additional optical components were placed into the excitation and emission beam path. Dielectric bandpass filters (Omega, Brattleboro, VT) reduced the out-of-band light of the excitation monochromator and long pass filters (colored glass filters, Schott Glass Technologies, Duryea, PA) prevented stray light generated in the sampling chamber from reaching the detector. For each of the three excitation wavelengths a different filter set was used. At 290 nm excitation, emission spectra were recorded from 300 to 570 nm, at 365 nm excitation from 375 to 720 nm emission, and at 460 nm excitation from 470 to 800 nm emission. In all measurements at 290 nm excitation, emission peaks were observed at 340 nm consistent with tryptophan. At 365 nm excitation emission peaks were located at 450 nm consistent with NAD(P)H and at 420 nm consistent with water Raman scattering. At 460 nm excitation, emission peaks were located at 520 nm consistent with FAD emission and at 540 consistent with water Raman scattering. Studies were conducted using a microcuvette with a volume of 200 μL. The cells were repeatedly stirred with a pipette to prevent settling between the measurements. A fluorescence standard was measured each measurement day at all three excitation wavelengths to monitor system drift. The standard solution was 2 mg/L Rhodamine 610 (Exciton, Dayton, OH) in ethylene glycol. 2.1.3.Cell Treatment4-HPR was obtained from Sigma Chemicals (St. Louis, MO). A 10 mM stock solution was made in 100 DMSO and stored at −20 °C. Recombinant human TGF-β1 was obtained from R&D Systems (Minneapolis, MN). A 10 μg/mL stock solution was made using 4 mM HCL containing 1 mg/ml BSA (HCL-BSA) and stored at −70 °C. Immediately prior to use, 4-HPR (2, 5, and 10 μM) and TGF-β1 (10 ng/mL) stock solutions were diluted in culture medium, 106 exponentially growing cells were incubated for three days with the different concentrations of 4-HPR (2, 5, 10 μM/mL) and/or TGF-β1 (10 ng/ml). 2.1.4.DNA Fragmentation AssayCells were harvested after three days of treatment and analyzed for the presence of DNA fragments using the APO-DIRECT Kit (Phoenix-Flow Systems, Inc., San Diego, CA). Briefly, this involves terminal deoxynucleotidyl transferase [TdT]-mediated (TUNEL) labeling of the 3′-hydroxyl ends of DNA fragments formed during apoptosis with fluorescein-tagged dUTP which reveals apoptosis using flow cytometry. 2.1.5.Cell Survival (Growth Inhibition) AssayCells were seeded in 96 well dishes at densities ranging from 1000 to 3000 cells per well and allowed to attach overnight. The exponentially growing cells were treated in triplicate with TGF-β1(10 μg/mL), 4-HPR (2, 5, 10 μg) or medium alone for three days. Cell numbers were estimated using a modified sulforhodamine B (SRB) assay. A 0.4 (w/v) solution of SRB (Sigma Chemicals, St. Louis, MO) was made in 1 acetic acid. The medium was aspirated and the cells fixed in situ with 100 μL per well of 10 TCA for 60 min. at 4 °C. The plates were rinsed five times with de-ionized water and air dried. The plates were incubated for 10 min at room temperature with 50 μL per well of SRB. Unbound SRB was solubilized with 100 μL per well of unbuffered 10 mM Tris base. The optical densities were determined using a microtiter plate reader set at 492 nm. Percent cell survival was calculated and ID 50 was determined by inspection of dose response curves. 2.1.6.Data AnalysisFor further analysis of the spectra, intensities were measured at 290 nm excitation, 340 nm emission, at 365 nm excitation, 420 nm emission, and at 460 nm excitation, 520 nm emission. These locations correspond to tryptophan, NAD(P)H and FAD emission and lay outside the range of Raman scattering of water. The emission from the supernatant of the third wash was subtracted from all other measurements as the background. Redox ratio was then calculated. Growth inhibition, apoptosis, and fluorescence intensities at the three wavelengths and the resultant redox ratios were then evaluated statistically using the Spearman rank correlation test to evaluate correlation between variables. Interactions were explored using regression analysis. The studies that included TGF-β1 were included with the 4-HPR group either 0, 2, 5, or 10 μM 4-HPR for analysis because there was consistently no effect from TGF-β1 on FAD, NAD(P)H, cell survival or apoptosis; the correlation was 0. 2.2.Primate2.2.1.Experimental DesignEighteen female adult rhesus macaques were used in this exploratory study. This protocol was approved by the Animal Care and Use Committee at The University of Texas M.D. Anderson Cancer Center and was conducted at the Department of Veterinary Sciences in Bastrop, Texas, where all animals were caged separately. The animals were given 4-HPR (four monkeys), OCP (five monkeys), the combination of 4-HPR+OCP (five monkeys), or no medication (four monkeys) daily for three months. Doses of 4-HPR and OCP were calculated by allometric scaling26 and given orally. The OCP used was Ortho-Novum 1/35, a medium-dose oral contraceptive with 1 mg norethindrone and 35 μg ethinyl estradiol in each pill. The 4-HPR dose was calculated in the same manner from the accepted human dose of 200 mg daily. Prior to starting medication and following 90 days of medication, monkeys underwent laparotomy, spectroscopy, and ovarian biopsies. 2.2.2.FluorescenceFluorescence excitation-emission matrices (EEMs), which contain the fluorescence intensity as a function of both excitation and emission wavelengths, were measured. The spectroscopic system for in vivo use records EEMs in less than 1.5 min and consists of a xenon arc lamp coupled to a scanning spectrometer that provides excitation light. A fiberoptic probe directs excitation light to the tissue, collects emitted fluorescence light, and delivers it to an imaging spectrograph and charge coupled device camera. The interrogated tissue area is 2 mm in diameter. Fluorescence emission spectra ranging from 320 to 850 nm were collected sequentially at 19 excitation wavelengths ranging from 300 to 480 nm in 10 nm steps. Before assembling the data into fluorescence EEMs, system dependent response and background signals were removed. Tissue exposure to broadband UV radiation from this device is below the total exposure limits developed by the American Conference of Governmental Industrial Hygienists (ACGIH) for epithelial tissues. Initially the left ovary was optically interrogated and biopsied. Following three months of drug, the right ovary was optically interrogated and biopsied. Base line fluorescence measurements were collected from the contra lateral ovary (the ovary that was not biopsied). Only one ovary was biopsied due to the concern that a biopsy would affect the subsequent fluorescence measurement due to collagen formation as the ovary healed. 2.2.3.Data AnalysisFrom the fluorescence measurements, values were extracted which may relate to NAD(P)H and FAD fluorescence. Signals were averaged at 450 nm excitation and 535 nm emission which is consistent with FAD and collagen emission and at 365 nm excitation and 450 nm emission which is consistent with NAD(P)H and collagen emission NAD(P)H. Although these wavelength ranges include contributions from both structural proteins as well as FAD and NAD(P)H, drugs should only modulate cell fluorescence and not collagen matrix. Therefore, we expect changes to be attributable to the effect of the drugs on the epithelial cells or stromal cells in the regions of NAD(P)H and FAD, rather than to an effect on the collagen matrix, because these drugs are receptor mediated and should not affect collagen. However, both epithelial and stromal cells may be affected. For each monkey, data was available from three measurement sites. The three spectra were averaged yielding between four and five averaged measurements for each drug group. Using this information, we compared all fluorescence measurements between the first and second measurements using the two-tailed Student’s t test. Group means and standard errors were calculated and plotted. From the three sites per measurement, two were biopsied on the same ovary pre drug treatment and two were biopsied posttreatment on the opposite ovary. This allowed us to visually compare posttreatment data from ovaries which had been previously biopsied and ovaries which had not been biopsied. 3.Results3.1.Fluorescence Measurements3.1.1.CellsThe Spearman rank correlation test was used to determine the correlation between apoptosis, growth inhibition, and fluorescence measurements. The presence or absence of TGF-β1 did not affect either survival, apoptosis, or redox ratio in this set of experiments (data not shown). Results varied between cell lines. There is a strong correlation between redox ratio and cell survival (p=0.0274), FAD and apoptosis (p<0.001), and redox ratio and apoptosis (p=0.0045) in the regression model (Table 1). There is consistent interaction with the cell line in the regression model, suggesting a different response with the different cell types. NOE cells showed a strong correlation between NAD(P)H fluorescence intensity and survival (p=0.04), between redox ratio and survival (p=0.018), and redox ratio and NADH fluorescence intensity (p=0.005). Figure 1 shows the relationship between cell survival and redox ratio in the three cell lines. Redox ratio increased in all three cell lines as cell survival decreased, (or growth inhibition increased). As the dose of 4-HPR increased, the cell survival decreased in all three experiments, but the sensitivity of the cells varied. IOSE 261 showed a slightly different response. The association between NAD(P)H fluorescence intensity and survival (p=0.02) persisted, but there was not an association with the other variables. IOSE 29 showed a correlation between NAD(P)H fluorescence intensity and survival (p<0.0001), between NAD(P)H fluorescence intensity and apoptosis (p=0.0002), FAD and apoptosis (p=0.04), redox ratios and survival (p=0.0002), and redox ratio and NAD(P)H fluorescence intensity (p=0.0001), suggesting that IOSE 261 is more sensitive to 4-HPR than IOSE 29. Figure 1Cell survival compared to redox potential in three cell lines NOE (a), IOSE 261 (b), and IOSE 29 (c). Increasing doses of 4-HPR show a decreased rate of cell survival. ![]() Table 1
Percent apoptosis, as visualized in Figure 2, varied between cell lines and dose of 4-HPR. NOE and IOSE 261, respectively, did not show significant changes in apoptosis with 2 and 5 μM 4-HPR. However, they still showed an increase in redox ratio with increasing doses of 4-HPR. The IOSE 29 did have an increase in apoptosis at the 5 μM dose which correlated with an increase in redox ratio. The 10 μM dose of 4-HPR was used on this cell line and induced a higher apoptosis than the 5 μM dose. The higher rate of apoptosis correlated with a higher redox ratio. Both apoptotic rates and redox ratios varied between cell lines which was consistent with different sensitivities of the cell lines to 4-HPR. 3.1.2.PrimatesConsistent differences in the absolute fluorescence intensities and relative contributions were noted between the pre- and postdrug measurements in each drug group as well as the controls. The advantage of this model is that variability due to time can be evaluated as well as variability due to drug effect. The differences observed in the control group were much smaller than those seen in the three groups receiving drugs. Changes observed in the control group could be attributed to natural fluctuations of the optical signal. When the average change within each group was compared, the NAD(P)H related signal decrease was statistically significant for the OCP (p=0.02) and the combination group (OCP+4-HPR) compared to the control group (p=0.01) [Figure 3(a)]. In the 4-HPR and control group, this signal increased but the change in the 4-HPR was not statistically different from the control group. The largest increase in the FAD related signal was found in the combination group and the 4-HPR group [Figure 3(b)]. The standard deviation was large and the number of samples small, yielding p values too large to be significant. However, the greatest effect was found in the 4-HPR group. Figure 3(a) Compares NADH increase between three drug groups and control group, OCP (p=0.02) and combination p=0.01 significant when compared to control group. (b) Compares FAD increase between drug groups and control group, p values not significant. (c) Compares redox potentials between drug groups and control group, p values not significant. ![]() Redox ratios increased in each group [Figure 3(c)]. The change in the control group was the smallest. The 4-HPR group changed the redox potential due to its contribution from increasing FAD, while the OCP group changed the redox potential due to its relative decrease in NADH. The combination group showed contribution from both increased FAD and decreased NADH. Although these differences did not achieve statistical significance related to small numbers within groups and large variances, there appears to be a trend of increasing redox potentials with both drugs. The visual comparison of data from ovaries that had been previously biopsied to data from ovaries which had not been biopsied showed no noticeable difference. This implies that healing response did not dramatically alter fluorescence. 4.DiscussionFluorescence spectroscopy is being used to detect cancers noninvasively in many organ systems.17 18 19 20 24 In this study, we evaluated it as a method to detect drug activity in the ovary in primates and to measure metabolic activity in human cell culture. In our prior pilot study in women22 differences in EEMs were found in the area of NAD(P)H fluorescence and hemoglobin absorption. These changes were attributed to increased NAD(P)H and hemoglobin presence. In tumors, NADH and FADH are postulated to increase as a result of alterations in blood flow, decreased pH of the tissue, and abnormal mitochondria as well as abnormal transport of electron carrier molecules into the mitochondria28 29 where the electron transport takes place. Intriguingly, this study suggests that these chemopreventive agents alter the metabolism of normal tissue in a direction opposite to that of cancer. TGFβ has been found to induce apoptosis in ovarian cancer cell lines and has been hypothesized to be a primitive surveillance mechanism for abnormal cells.25 However, data from our laboratory have shown inconsistent results with TGFβ and in this study it had no effect. Limitations of the cell data include the lack of interaction of epithelial cells with the stroma, which may account for the differences seen between primate ovaries and the isolated human ovarian epithelial cells. They both, however, are strongly associated with an increased redox ratio. No cell line can approximate the behavior of tissue in vivo in humans, but mechanistic studies can be done on cells to understand the potential application to in vivo systems. The cell line data suggest that redox ratios are useful to evaluate biologic activity of these drugs. Limitations of our primate study include too few numbers to reach significance due to large inter-animal variances; however, there appears to be a relationship between increasing redox ratios and treatment with 4-HPR and OCP. Effects of 4-HPR and OCP appear additive or potentially synergistic when the two drugs are combined, consistent with different mechanisms of action for these drugs. The primate results also suggest that redox values may correlate with drug effects. The 4-HPR activity in the mitochondria may be evaluated with fluorescence spectroscopy, because natural fluorophores in cells, NAD(P)H and FAD are affected by 4-HPR. Although the mechanism of the OCP prevention of ovarian cancer is poorly understood, and has not yet been validated in the laboratory, there is a different signal seen with the monkeys receiving 4-HPR and those receiving OCP. Use of both the cell model and the primate model are powerful tools for studying the biologic activity of these drugs. Areas for future research include a study with larger numbers of monkeys to attain additional statistical significance as well as further laboratory work to elucidate the mechanism of OCP and 4HPR activity in cell culture. Ultimately, this work will be extended to monitoring clinical trials in chemoprevention. REFERENCES
G. Kelloff
,
C. W. Boone
,
J. A. Crowell
,
S. G. Nayfield
,
E. Hawk
,
V. A. Steele
,
R. A. Lubet
, and
C. C. Sigman
,
“Strategies for phase II cancer chemoprevention trials: Cervix, endometrium, and ovary,”
J. Cell Biochem. Suppl. , 23 1
–9
(1995). Google Scholar
K. Dhingra
,
“A phase II chemoprevention trial design to identify surrogate endpoint biomarkers in breast cancer,”
J. Cell Biochem. Suppl. , 23 19
–24
(1995). Google Scholar
G. De Palo
,
U. Veronesi
,
T. Camerini
,
F. Formelli
,
G. Mascotti
,
C. Boni
,
V. Fooser
,
M. Del Vecchio
,
T. Campa
, and
A. Costa
,
“Can fenretinamide protect women against ovarian cancer,”
J. Natl. Cancer Inst. , 87 146
–147
(1995). Google Scholar
S. Y. Sun
,
J. M. Kurie
,
P. Yue
,
M. I. Dawson
,
B. Shroot
,
R. A. S. Chandraratna
,
W. K. Hong
, and
R. Lotan
,
“Differential responses of normal, premalignant and malignant human bronchial epithelial cells to receptor-selective retinoids,”
Clin. Cancer Res. , 5 431
–437
(1999). Google Scholar
S. Y. Sun
,
W. Li
,
P. Yue
,
S. M. Lippman
,
W. K. Hong
, and
R. Lotan
,
“Mediation of N-(4-Hydoxyphenyl) retinamide-induced apoptosis in human cancer cells by different mechanisms,”
Cancer Res. , 59 2943
–98
(1999). Google Scholar
D. D. Taylor
,
C. G. Taylor
,
P. H. Black
,
C. G. Jiang
, and
I. N. Chou
,
“Alterations of cellular characteristics of a human ovarian teratocarcinoma cell line after in vitro treatment with retinoids,”
Differentiation , 43 123
–130
(1990). Google Scholar
A. H. Wyllie
,
“Apoptosis and carcinogenesis,”
Eur. J. Cell Biol. , 73 189
–197
(1997). Google Scholar
R. Dabal
,
C. M. Boyer
,
A. Berchuck
,
A. Roberts
,
N. Roche
,
M. Sporn
, and
R. Bast
,
“Synergistic inhibition of ovarian cancer cell proliferation by TGFβ and retinoic acid (RA) derivatives,”
Proc. American Association of Cancer Research , 36 635
(1995). Google Scholar
R. Supino
,
M. Crosti
,
M. Clerici
,
A. Warlters
,
L. Cleris
,
F. Zunino
, and
F. Formelli
,
“Induction of apoptosis by fenretinide (4-HPR) in human ovarian carcinoma cells and its association with retinoic acid receptor expression,”
Int. J. Cancer , 65 491
–497
(1996). Google Scholar
X. Liu
,
C. N. Kim
,
J. Yang
,
R. Jemmerson
, and
X. Wang
,
“Requirement for dATP and cytochrome c,”
Cell , 86 147
–157
(1996). Google Scholar
S. Suzuki
,
M. Higuchi
,
R. J. Proske
,
N. Oridate
,
W. K. Hong
, and
R. Lotan
,
“Implication of mitochondria-derived reactive oxygen species, cytochrome C and caspace-3 in N-(4-Hydroxyphenyl) retinamide-induced apoptosis in cervical carcinoma cells,”
Oncogene , 18 6380
–6387
(1999). Google Scholar
A. S. Whittemore
,
R. Harris
,
J. Itnyre
, and Collaborative Ovarian Cancer Group,
“Characteristics relating to ovarian cancer risk: Collaborative analysis of 12 U.S. case-control studies. II. Invasive epithelial ovarian cancers in white women,”
Am. J. Epidemiol. , 136 1184
–1203
(1992). Google Scholar
S. Franceschi
,
F. Parazzini
,
E. Negri
,
M. Booth
,
C. La Vecchia
,
V. A. Beral
, and
D. Trichopoulos
,
“Pooled analysis of 3 European case-control studies. III. Oral contraceptive use,”
Int. J. Cancer , 49 61
–65
(1991). Google Scholar
J. Rosenberg
,
S. Shapiro
,
D. Slone
,
D. W. Kaufman
,
S. P. Helmrich
,
O. S. Miettinen
,
P. D. Stolley
,
N. B. Rosenshein
,
D. Schottenfeld
, and
R. L. Engle
,
“Epithelial ovarian cancer and combination oral contraceptives,”
J. Am. Med. Assoc. , 243
(23), 3210
–3212
(1982). Google Scholar
R. Marchesini
,
M. Brambilla
,
E. Pignoli
,
G. Bottiroli
,
A. C. Croce
,
M. Dal Fante
,
P. Spinelli
, and
S. di Palma
,
“Light-induced fluorescence spectroscopy of adenomas, adenocarcinmas and non-neoplastic mucose in human colon,”
Photochem. Photobiol. , 14
(3), 219
–230
(1992). Google Scholar
R. M. Cothren
,
R. R. Richards-Kortum
,
R. P. Rava
,
G. A. Boyce
,
M. Doxtader
,
R. Blackman
,
T. B. Ivanc
, and
G. B. Hayes
,
“Gastrointestinal tissue diagnosis by LIF spectroscopy at endoscopy,”
Gastrointest. Endosc. , 36 105
–111
(1990). Google Scholar
J. Hung
,
S. Lam
,
J. C. LeRiche
, and
B. Palcic
,
“Autofluorescence of normal and malignant bronchial tissue,”
Lasers Surg. Med. , 11
(2), 99
–105
(1991). Google Scholar
S. Lam
,
J. Y. C. Hung
,
S. M. Kennedy
,
J. C. LeRiche
,
R. Vedal
,
B. Nelems
,
C. E. Macaulay
, and
B. Palcic
,
“Detection of dysplasia and carcinoma in situ by ratio fluorometry,”
Am. Rev. Respir. Dis. , 146 1458
–1461
(1992). Google Scholar
B. Chance
,
“Metabolic heterogeneities in rapidly metabolizing tissues,”
J. Appl. Cardiol. , 4 207
–221
(1989). Google Scholar
P. A. Kruk
,
S. L. Maines-Bandiera
, and
N. Auersperg
,
“A simplified method to cuture human ovarian surface epithelium,”
Lab. Invest. , 63 132
–136
(1990). Google Scholar
G. C. Rodriguez
,
D. K. Walmer
,
M. Cline
,
H. Krigman
,
B. A. Lessey
,
R. S. Whitaker
,
R. Dodge
, and
C. L. Hughes
,
“Effect of progestin on the ovarian epithelium of macaques: Cancer prevention through apoptosis?,”
J. Soc. Gynecol. Investig. , 5 271
–276
(1998). Google Scholar
A. Berchuck
,
G. Rodriquez
,
G. Olt
,
R. Whitaker
,
M. Boente
,
B. Arrick
,
D. Clarke-Pearson
, and
R. Bast
,
“Regulation of growth of normal epithelial cells and ovarian cancer cell lines by transforming growth factor-β,”
Am. J. Obstet. Gynecol. , 166 676
–684
(1992). Google Scholar
A. Gillenwater
,
R. Jacob
, and
R. Richards-Kortum
,
“Fluorescence spectroscopy: A technique with potential to improve the early detection of aerodigestive tract neoplasia,”
Head Neck , 20 556
–562
(1998). Google Scholar
C. J. Gulledge
and
M. W. Dewhirst
,
“Tumor oxygenation: A matter of supply and demand,”
Anticancer Res. , 16 741
–750
(1996). Google Scholar
|
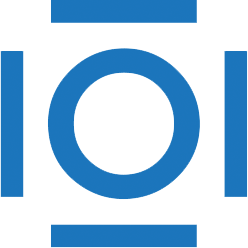
CITATIONS
Cited by 33 scholarly publications.
Luminescence
Cell death
Cancer
Ovarian cancer
Ovary
Fluorescence spectroscopy
Control systems