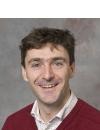
Linear spectroscopic inversions, in which photoacoustic amplitudes are assumed to be directly proportional to absorption coefficients, are widely used in photoacoustic imaging to estimate blood oxygen saturation because of their simplicity. Unfortunately, they do not account for the spatially varying wavelength-dependence of the light fluence within the tissue, which introduces “spectral coloring,” a potentially significant source of error. However, accurately correcting for spectral coloring is challenging, so we investigated whether there are conditions, e.g., sets of wavelengths, where it is possible to ignore the spectral coloring and still obtain accurate oxygenation measurements using linear inversions. Accurate estimates of oxygenation can be obtained when the wavelengths are chosen to (i) minimize spectral coloring, (ii) avoid ill-conditioning, and (iii) maintain a sufficiently high signal-to-noise ratio (SNR) for the estimates to be meaningful. It is not obvious which wavelengths will satisfy these conditions, and they are very likely to vary for different imaging scenarios, making it difficult to find general rules. Through the use of numerical simulations, we isolated the effect of spectral coloring from sources of experimental error. It was shown that using wavelengths between 500 nm and 1000 nm yields inaccurate estimates of oxygenation and that careful selection of wavelengths in the 620- to 920-nm range can yield more accurate oxygenation values. However, this is only achievable with a good prior estimate of the true oxygenation. Even in this idealized case, it was shown that considerable care must be exercised over the choice of wavelengths when using linear spectroscopic inversions to obtain accurate estimates of blood oxygenation. This suggests that for a particular imaging scenario, obtaining accurate and reliable oxygenation estimates using linear spectroscopic inversions requires careful modeling or experimental studies of that scenario, taking account of the instrumentation, tissue anatomy, likely sO2 range, and image formation process.
Since it was first demonstrated more than a decade ago, the single-pixel camera concept has been used in numerous applications in which it is necessary or advantageous to reduce the channel count, cost, or data volume. Here, three-dimensional (3-D), compressed-sensing photoacoustic tomography (PAT) is demonstrated experimentally using a single-pixel camera. A large area collimated laser beam is reflected from a planar Fabry–Pérot ultrasound sensor onto a digital micromirror device, which patterns the light using a scrambled Hadamard basis before it is collected into a single photodetector. In this way, inner products of the Hadamard patterns and the distribution of thickness changes of the FP sensor—induced by the photoacoustic waves—are recorded. The initial distribution of acoustic pressure giving rise to those photoacoustic waves is recovered directly from the measured signals using an accelerated proximal gradient-type algorithm to solve a model-based minimization with total variation regularization. Using this approach, it is shown that 3-D PAT of imaging phantoms can be obtained with compression rates as low as 10%. Compressed sensing approaches to photoacoustic imaging, such as this, have the potential to reduce the data acquisition time as well as the volume of data it is necessary to acquire, both of which are becoming increasingly important in the drive for faster imaging systems giving higher resolution images with larger fields of view.
The goal in PAT is to form an image of the absorbed optical energy density field via acoustic inverse problem approaches from the measured ultrasound data. Quantitative PAT (QPAT) proceeds from these images and forms quantitative estimates of the optical properties of the target. This optical inverse problem of QPAT is illposed. To alleviate the issue, spectral QPAT (SQPAT) utilizes PAT data formed at multiple optical wavelengths simultaneously with optical parameter models of tissue to form quantitative estimates of the parameters of interest.
In this work, the inverse problem of SQPAT is investigated. Light propagation is modelled using the diffusion equation. Optical absorption is described with chromophore concentration weighted sum of known chromophore absorption spectra. Scattering is described by Mie scattering theory with an exponential power law. In the inverse problem, the spatially varying unknown parameters of interest are the chromophore concentrations, the Mie scattering parameters (power law factor and the exponent), and Gruneisen parameter. The inverse problem is approached with a Bayesian method. It is numerically demonstrated, that estimation of all parameters of interest is possible with the approach.
The optical and acoustic properties of PVCP can be tuned to be broadly representative of soft tissue. The Grüneisen parameter is larger than expected in tissue, which is an advantage as it increases the signal-to-noise ratio of the photoacoustic measurements. Interestingly, when the absorption was altered by adding absorbers, the absorption spectra measured using high peak power nanosecond-pulsed sources (typical in photoacoustics) were repeatably different from the ones measured using the low power source in the spectrophotometer, indicative of photochemical reactions taking place.
View contact details